Return
to Table of Contents Return to main
menu
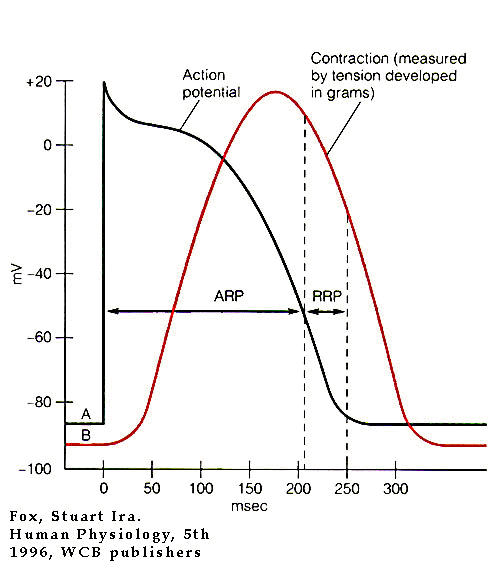
Return
to Table of Contents Return to main
menu
Return
to Table of Contents Return to main
menu
Different Forms of
Cardiac Action Potential
slightly modified from: Crawford, M. H. and
DiMarco, J. P, Cardiology, Mosby, St. Louis,
MO. 2001
Electrophysiology: Pacemaker and Cardiac Cells
American Association of Critical-Care Nurses (AACN)
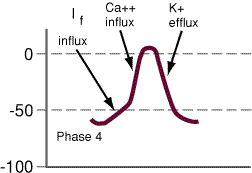 |
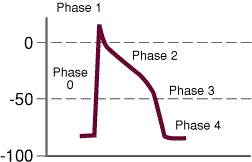 |
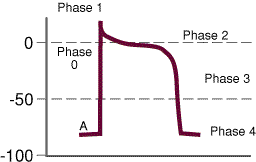 |
(left to right: pacemaker cell, atrial
muscle cell, ventricular muscle cell)
Figure by: Barbara McLean, MN, RN, CCRN, CRNP
Clinical Specialist in Critical Care, Grady Health System
Lecturer, Emory University School of Medicine
Clinical Faculty, Nell Hodgson Woodruff School of Nursing, Emory University
Clinical Associate, Morehouse School of Medicine
Atlanta, Georgia
Ventricular
Muscle Cell Action Potential
- Phase 0:
- Activation of
fast Na+ channel-- initial
depolarization; slope & magnitude of a 0 will be
dependent on the resting membrane potential (A in the
diagram on the right)
- Phase 1:
- Partial repolarization; K+ efflux
- Phase 2:
- Ca2+ entry
with continued K+ efflux =
"plateau phase". Initial Ca2+
influx through slow L- type Ca2+ channels
initiates further Ca2+ release from
and sarcoplasmic reticulum stores: Free Ca2+
binds to contractile proteins (e.g. troponin C)
promoting/enhancing muscle contraction
- catecholamines (sympathomimetic amines
e.g. epinephrine, norepinephrine (Levophed)) increase
slow-inward Ca2+ currents-- a
mechanism by which sympathomimetic agents enhance
inotropism
- Phase 3
- This phase is dominated by K+
efflux, i.e. repolarization. The
membrane potential moves towards the original resting
level. Phase 3 ccorresponds to the effective/absolute
refractory period.
- Restoration of ionic gradients to
"pre-action potential" levels requires the
action of the Na+/K+
membrane ATPase-dependent transporter
- Phase 4
- This phase is between action
potentials. In some cell types, phase 4 depolarization
(diastolic depolarization) can occur {especially, for example in
"pacemaker" cells}.
|
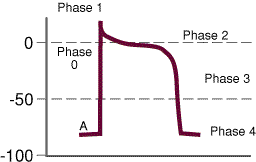 |

Cardiac Conduction System: Review
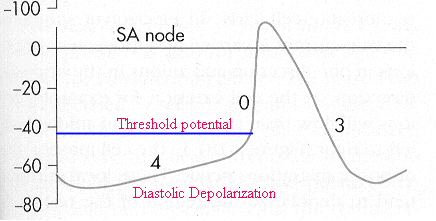
Adapted from Berne and Levy (Berne, R.M and
Levy, M. N. Cardiovascular Physiology,8th Edition, Mosby, St.
Louis, Mo. 2001 (Fig. 2-17)
-
SA & AV nodal, slow response,
fibers exhibit Ca2+-mediated inward
currents which are responsible for depolarization
-
The channel type appears to be of
the L-type Ca2+channel,
voltage-gated, typically activated membrane potentials > -40
mV
-
L-type calcium channel
inactivation is dependent on (a) cell membrane potential, [ Ca2+],
and time
-
L-type calcium channel
antagonists include:
Benzothiapines (e.g.
diltiazem (Cardiazem))
Dihydropyridines (e.g.
nifedipine (Procardia, Adalat) & nitrendipine
Phenylalkylamines (e.g.
verapamil (Isoptin, Calan)
Left Ventricular Tachycardia
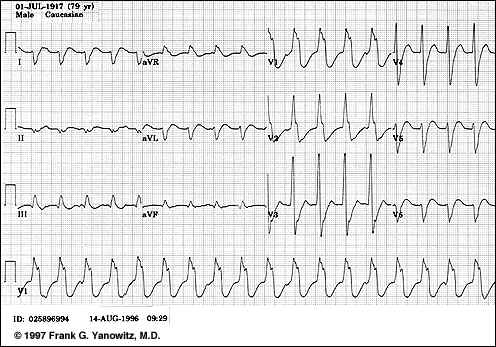
-
"Several features confirm this wide QRS tachycardia to be ventricular in origin. The morphology of the QRS in V1 has a distinct notch on the downstroke making it
highly unlikely to be RBBB aberration. The QRS is entirely negative in lead V6. The frontal plane QRS axis is +150. The direction of ventricular activation is from
left to right and posterior to anterior, suggesting a left ventricular origin"
Frank G. Yanowitz, M.D. Copyright 1998 used with permission
Return
to Table of Contents Return to main
menu
-
Internodal
pathway
- Three internodal tracts provide input to
the AV node {i.e., primary function is transmission of SA
nodal pacemaker impulses to the AV node}
- Internodal pathways are localized in the
inter-atrial septum and right atrial walls
- Three functional AV nodal zones:
- AN zone: joining atrium to the node
- N (nodal) region
- NH zone: joining the node to the bundle
of His
- Significance: functional AV nodal zones:
- Delay between atrial impulse
transmission to distal conduction components-- due to
"N" regions & "AN" regions
- This delay allows for adequate
ventricular filling subsequent to atrial contraction
{associated with the PR ECG interval}
- Structure: AV node
- Button-shaped structure (22 x 10 x 3 mm)
- Location:
- Right posterior side of interatrial septum
near coronary sinus ostium (coronary sinus = largest
cardiac vein), near the tricuspid valve septal leaflet
- Embryological development -- left-sided,
accounting for left-sided domination of AV nodal vagal
innervation
- Function: reduces atrial-ventricular
conduction, thus allowing time for ventricular optimal filling
- AV node blood supply provided by the right
coronary artery
Return
to Table of Contents Return to main
menu
-
Bundle
of His:
-
AV nodal fibers proceed to the
bundle of His
-
Bundle of His extends
subendocardially along the right side of the interventricular
septum, then divides, continuing as the right bundle branch
-
Singular communication pathway
between atria and ventricles
-
The left bundle branch also
arises from the bundle of His, also passing through the
interventricular septum and further dividing into a thin
anterior fascicle as well as a thick posterior fascicle
proceedings subendocardially along the left side of the
interventricular septum. (see diagram below)
-
Left bundle branch (LBB):
-
Initiation point: end of the His
bundle
-
Progresses through the
interventricular septum
-
LBB fibers innervate:
-
the left ventricle
-
the interventricular septum
-
Cardiac regions innervated by the
LBB are the first to depolarize
-
Ending point: at the beginning of
the left anterior and left posterior fascicles (LAF & LPF)
-
The Purkinje fiber network
--deriving from the right bundle and the two left fascicles --
allows impulse conduction throughout the ventricle
-
Contraction sequence, as a result of
action potential propagation through Purkinje fibers
-
Interventricular myocardial
tissue (septum) & papillary muscle
-
Septal contraction provides an
"anchor" for the heart as the ventricular free wall
begins contracting and papillary muscle tightening prevents
tricuspid & mitral valve prolapse during early systole
-
Muscle fiber contraction proceeds
outward from the endocardial region to the epicardial surface
-
The right ventricle contracts
before the left ventricle secondary to differences in wall
thickness {differences in output from the left vs. right-side
are compensated in accord with Starling's Law}
Automaticity
-
Overview
-
Automaticity, a SA nodal
normal characteristic and a characteristic of specialized
His-Purkinje system fibers as well as some specialized atrial
fibers is defined as a cardiac cellular property which causes
spontaneous cell membrane depolarization.
-
This depolarization occurs during
phase 4 of the action potential.
-
The primary characteristic of
automaticity is that the resting membrane potential is not
stable but rather decreases (towards 0 millivolts (mv)) until
threshold is reached an action potential generated
-
In those normal cells which would
be expected to exhibit automaticity, specific ionic currents
have been identified as responsible. These currents are:
-
an inward current (If),
probably carried by Na+ which
tends to depolarize the membrane
-
a second inward current
carried by Ca2+, (ICa2+),
which is also depolarizing
-
and a third outward current
carried by K+ (IK+),
the conductance of which tends to decrease during phase 4,
those leading to a net depolarizing effect. This outward
current tends to oppose the inward (If)
and (ICa2+) currents'
depolarizing influence. Thus the time-dependent
decrease in IK+ is an important factor
leading to the positive slope associated with phase 4
depolarization.
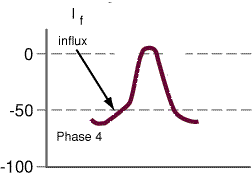
Adapted from Berne, R.M and Levy, M. N. Cardiovascular Physiology,8th Edition,
Mosby, St. Louis, Mo., p. 30, Figure 2-23, 2001
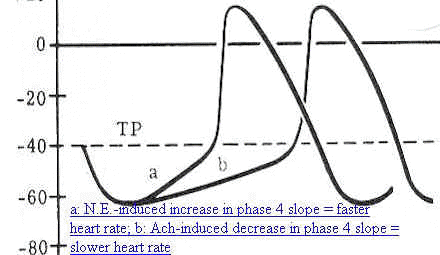
Adapted from: Blanck, Thomas J.J. and Lee, David L, Cardiac Physiology, in Anesthesia, 5th edition,vol
1, (Miller, R.D, editor;
consulting editors, Cucchiara, RF, Miller, Jr.,ED, Reves, JG,
Roizen, MF and Savarese, JJ) Churchill Livingston, a Division of
Harcourt Brace & Company, Philadelphia, p 626, 2000- primary
source: Berne & Levy Electrical Activity of the Heart, In
Cardiovascular Physiology, 4th ed, St. Louis, CV Mosby, 1981, p.5
Factors that Increase Automaticity:
hypokalemia1 |
cardiac
fiber stretch2 |
beta-adrenergic
receptor activation
|
injury currents3
|
acidosis4 |
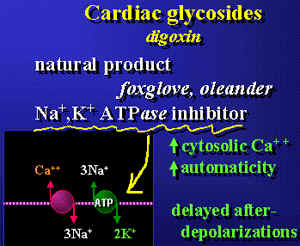
Cardiovascular pharmacology module
by Lee, EJD {see for more information: http://www.med.nus.edu.sg/phar/medlect/inotropes.htm)}
Both early and delayed
afterdepolarizations are probably Ca2+
dependent
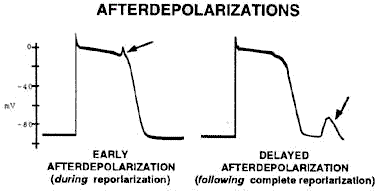
-
2 Stretch-induced
depolarizations are known to cause
arrhythmias. Furthermore stretch-activated ion channel have
been identified and their electrophysiological properties
characterized. Mechanosensitive ion channels were first discovered
in skeletal muscle with subsequent identification in cardiac cells,
bone, and in most other types of cells including those in bacteria
and plants (see Sachs F: 1992.
Stretch sensitive Ion channels: an update. In Corey DP, Roper SD,
eds. Sensory Transduction, New York, Rockefeller University
press,Soc Gen Physiol. pp 241-260 and more recently GCL Bett and F.
Sachs (1997) Cardiac Mechanosensitivity and Stretch-Activated Ion
Channels, Trends in Cardiovascular Medicine 7: 4-8)
-
3 Injury Currents:
Consider the 12-lead ECG: During an antianginal episode, ST segment
depression may be observed. Normally the ST segment is
associated with phase 2 of the myocardial action potential.
Phase 2 is typically isoelectric (no significant membrane
potential change is occurring-see below)
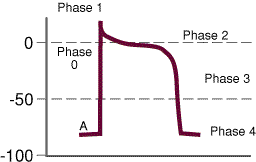
-
With ischemia, the resting
membrane potential in the ischemic region is reduced compared to
surrounding healthy tissue. As a consequence of this
membrane potential difference, current flows between the two
regions. This current is referred to as an injury current
and is reflected by deviations in the ECG ST segment
-
For subendocardial ischemia,
the ST vector is towards the ventricular cavity, resulting
in surface leads reporting ST depression; however, in
transmural ischemia, the ST vector is oriented towards the
surface lead, causing ST segmental elevation {Biomedical
Sciences 245B, Virginia Commonwealth University:
Pathophysiology of Disease: Ischemic Hard Disease
(Krishnan), http://wcb.ucr.edu/wcb/school/CNAS/bmsc/lloo/11/modiules/page21.html)}
|